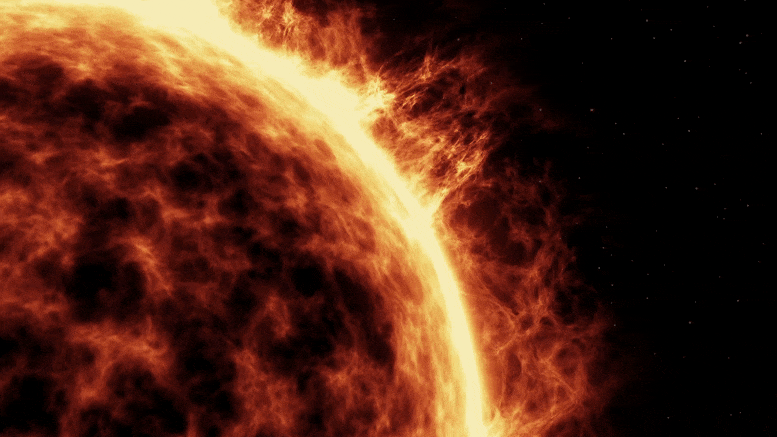
Astronomer har endelig løst konflikten mellom den indre strukturen til Solen som er bestemt fra soloscillasjoner og strukturen utledet fra den grunnleggende teorien om stjernenes evolusjon.
Nye beregninger av solspektrum løser tiår lang kontrovers om sammensetningen av stjernen vår.
Selv om solen vår er langt nærmere enn noen annen stjerne i universet, har den fortsatt sine mysterier. Tross alt er det fortsatt 93 millioner miles (150 millioner kilometer) fra jorden, og vi har bare et smalt perspektiv. Pluss at overflaten er blemmer varm, atmosfæren enda varmere, og at den konstant sprenger ut partikler med omtrent 1 million miles per time. Det er ikke rart at vi fortsatt gjør nye oppdagelser.
Faktisk har astronomer nettopp løst den tiår lange soloverflodskrisen: konflikten mellom den indre strukturen til solen som bestemt fra soloscillasjoner (helioseismologi) og strukturen avledet fra den grunnleggende teorien om stjernenes evolusjon, som igjen er avhengig av målinger av dagens sols kjemiske sammensetning. Nye beregninger av fysikken til solens atmosfære gir oppdaterte resultater for overflod av forskjellige kjemiske elementer, som løser konflikten. Spesielt inneholder solen mer oksygen, silisium og neon enn tidligere antatt. Metodene som brukes lover også betydelig mer nøyaktige estimater av den kjemiske sammensetningen av stjerner generelt.
Hva gjør du når en velprøvd metode for å bestemme solens kjemiske sammensetning ser ut til å være i strid med en nyskapende, presis teknikk for å kartlegge solens indre struktur? Det var situasjonen for astronomer som studerer solen – inntil nye beregninger som nå er publisert av Ekaterina Magg, Maria Bergemann og kolleger, og som løser den tilsynelatende motsetningen.

Spectrum of the Sun, tatt med NARVAL-spektrografen med svært høy oppløsning installert ved Telescope Bernard Lyot, Observatoire Midi-Pyrénées. Spektre som dette, spesielt egenskapene til de mørke absorpsjonslinjene som er godt synlige i dette bildet, lar astronomer utlede en stjernes temperatur og kjemiske sammensetning. Kreditt: © M. Bergemann / MPIA / [email protected]
Den velprøvde metoden det er snakk om er spektralanalyse. For å bestemme den kjemiske sammensetningen til vår sol, eller av en hvilken som helst annen stjerne der ute, går astronomer rutinemessig til spektre: den regnbuelignende dekomponeringen av lys i dets forskjellige bølgelengder. Stellarspektre inneholder iøynefallende, skarpe mørke linjer, først lagt merke til av William Wollaston i 1802, kjent gjenoppdaget av Joseph von Fraunhofer i 1814, og identifisert som avslørende tegn som indikerer tilstedeværelsen av spesifikke kjemiske elementer av Gustav Kirchhoff og Robert Bunsen på 1860-tallet.
Solfakta
- Type: G-type hovedsekvensstjerne (G2V)
- Uformell type: Gul dverg
- Alder: ~4,5 milliarder år
- Volum: 1,3 millioner ganger jordens
- Kjernetemperatur: 27 millioner grader[{” attribute=””>Fahrenheit (15 million degrees
Just like seismic waves provide geologists with crucial information about the Earth’s interior, or like the sound of a bell encodes information about its shape and material properties, helioseismology provides information about the interior of the Sun.
Highly accurate helioseismic measurements gave results about the Sun’s interior structure that were at odds with the solar standard models. According to helioseismology, the so-called convective region within our Sun where matter rises and sinks down again, like water in a boiling pot, was considerably larger than the standard model predicted. The speed of sound waves near the bottom of that region also deviated from the standard model’s predictions, as did the overall amount of helium in the Sun. To top it off, certain measurements of solar neutrinos – fleeting elementary particles, hard to detect, reaching us directly from the Sun’s core regions – were slightly off compared to experimental data, as well.
Astronomers had what they soon came to call a “solar abundances crisis,” and in search of a way out, some proposals ranged from the unusual to the downright exotic. Did the Sun maybe accrete some metal-poor gas during its planet-forming phase? Is energy being transported by the notoriously non-interacting dark matter particles?
The newly published study by Ekaterina Magg, Maria Bergemann and colleagues has managed to resolve that crisis, by revisiting the models on which the spectral estimates of the Sun’s chemical composition are based. Early studies of how the spectra of stars are produced had relied on something known as local thermal equilibrium. They had assumed that locally, energy in each region of a star’s atmosphere has time to spread out and reach a kind of equilibrium. This would make it possible to assign to each such region a temperature, which leads to a considerable simplification in the calculations.
But as early as the 1950s, astronomers had realized that this picture was oversimplified. Since then, more and more studies incorporated so-called Non-LTE calculations, dropping the assumption of local equilibrium. The Non-LTE calculations include a detailed description of how energy is exchanged within the system – atoms getting excited by photons, or colliding, photons getting emitted, absorbed or scattered. In stellar atmospheres, where densities are far too low to allow the system to reach thermal equilibrium, that kind of attention to detail pays off. There, Non-LTE calculations yield results that are markedly different from their local-equilibrium counterparts.
Maria Bergemann’s group at the Max Planck Institute for Astronomy is one of the world leaders when it comes to applying Non-LTE calculations to stellar atmospheres. As part of the work on her PhD in that group, Ekaterina Magg set out to calculate in more detail the interaction of radiation matter in the solar photosphere. The photosphere is the outer layer where most of the Sun’s light originates, and also where the absorption lines are imprinted on the solar spectrum.
In this study they tracked all chemical elements that are relevant to the current models of how stars evolved over time, and applied multiple independent methods to describe the interactions between the Sun’s atoms and its radiation field in order to make sure their results were consistent. For describing the convective regions of our Sun, they used existing simulations that take into account both the motion of the plasma and the physics of radiation (“STAGGER” and “CO5BOLD”). For the comparison with spectral measurements, they chose the data set with the highest available quality: the solar spectrum published by the Institute for Astro- and Geophysics, University of Göttingen. “We also extensively focused on the analysis of statistical and systematic effects that could limit the
“We found, that according to our analysis the Sun contains 26% more elements heavier than helium than previous studies had deduced,” explains Magg. In astronomy, such elements heavier than helium are called “metals.” Only on the order of a thousandth of a percent of all atomic nuclei in the Sun are metals; it is this very small number that has now changed by 26% of its previous value. Magg adds: “The value for the oxygen abundance was almost 15% higher than in previous studies.” The new values are, however, in good agreement with the chemical composition of primitive meteorites (“CI chondrites”) that are thought to represent the chemical make-up of the very early solar system.
When those new values are used as the input for current models of solar structure and evolution, the puzzling discrepancy between the results of those models and helioseismic measurements disappears. The in-depth analysis by Magg, Bergemann and their colleagues of how spectral lines are produced, with its reliance on considerably more complete models of the underlying physics, manages to resolve the solar abundance crisis.
Maria Bergemann says: “The new solar models based on our new chemical composition are more realistic than ever before: they produce a model of the Sun that is consistent with all the information we have about the Sun’s present-day structure – sound waves, neutrinos, luminosity, and the Sun’s radius – without the need for non-standard, exotic physics in the solar interior.”
As an added bonus, the new models are easy to apply to stars other than the Sun. At a time where large-scale surveys like SDSS-V and 4MOST are providing high-quality spectra for an ever greater number of stars, this kind of progress is valuable indeed – putting future analyses of stellar chemistry, with their broader implications for reconstructions of the chemical evolution of our cosmos, on a firmer footing than ever before.
Reference: “Observational constraints on the origin of the elements: IV. Standard composition of the Sun” by Ekaterina Magg, Maria Bergemann, Aldo Serenelli, Manuel Bautista, Bertrand Plez, Ulrike Heiter, Jeffrey M. Gerber, Hans-Günter Ludwig, Sarbani Basu, Jason W. Ferguson, Helena Carvajal Gallego, Sébastien Gamrath, Patrick Palmeri and Pascal Quinet, 20 May 2022, Astronomy & Astrophysics.
DOI: 10.1051/0004-6361/202142971